Planning a drip irrigation system
On this page
- Water quality
- Matching the system design to the soil and crop type
- Irrigation system design
- Maximum number of irrigation shifts allowed
- Choosing an emitter
- Drip line location
- Drip drainage
- Sub-surface drip irrigation for permanent horticulture
- Frost control and drip irrigation
- Heat control and drip irrigation
- Converting existing plants to drip irrigation
When planning a drip irrigation system, there are many factors to consider. The most important of these are covered in this fact sheet.
- water quality
- soil type and variation
- crop variety, rootstock, and likely root zone depth
- number of irrigation shifts and the ability to meet peak daily water requirements
- emitter selection, discharge and spacing
- proposed irrigation management program, such as pulsing or intensive fertigation practices
- surface or sub surface irrigation
- management issues such as frost and heat control
- converting mature plantings
Issues covered in adjoining fact sheets are:
- system maintenance and monitoring
- filtration
- fertigation
Water quality
System blockages are a key issue for drip irrigation. All irrigators, regardless of the irrigation method, should be aware of their irrigation water quality to ensure that it is compatible with the crop and soil. Drip irrigators need to be particularly aware of their water quality as some water sources can create system blockages.
The following water quality issues should be considered when determining if a water source is suitable for drip irrigation:
- pH
- Iron and manganese concentration
- Hardness
- Calcium carbonate saturation index
- Turbidity
For information on how water quality can affect the performance of pressurised irrigation systems, see the Farm Water Quality and Treatment fact sheet on the NSW Department of Primary Industries website. If there is uncertainty about existing water quality, a water analysis should be conducted. A full water analysis will provide a water quality baseline, also help identify the type and level of filtration and possible disinfestation practices needed for the water source.
Matching the system design to the soil and crop type
A soil survey is essential for understanding relevant soil characteristics for irrigation. It classifies soils based on texture and depth, providing valuable information for designing the irrigation system layout.
While the cost of a soil survey is typically small compared to the overall expense of a new drip system, it is a worthwhile investment. It is recommended to hire a soil survey specialist to conduct the survey (Figure 1).
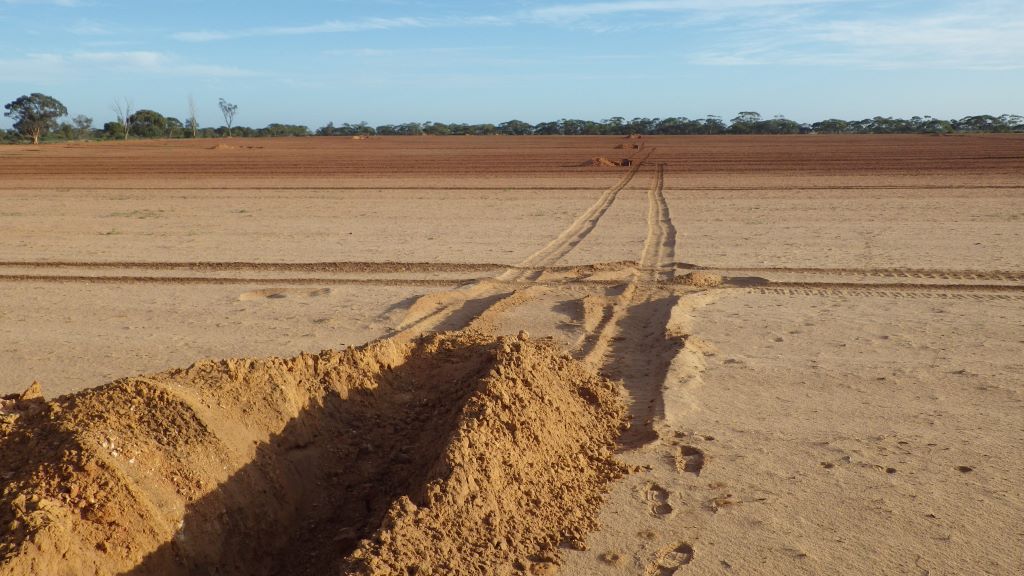
Figure 1. Soil surveys and associated irrigation and drainage management plans are standard practice before a new development. They should also be prepared before upgrading existing irrigation systems. Photo: Mallee CMA.
Understanding soil types is crucial for effective system planning. Soil pits reveal soil profiles (Figure 2), providing information on texture, depth, and water-holding capacity. Similar soil types allow similar rooting depths, dripper-wetted patterns, and drainage characteristics providing similar amounts of water to each plant. Wherever possible, the irrigation system should be designed so that similar soil types can be irrigated in the same shift.
A soil survey will also provide ripping, mounding and soil amelioration recommendations. These suggestions are most readily applicable in a new development or redevelopment situation but are also relevant in an existing orchard if drip conversions are proposed. Upgrading to drip irrigation from an existing sprinkler system will not solve inherent soil problems.
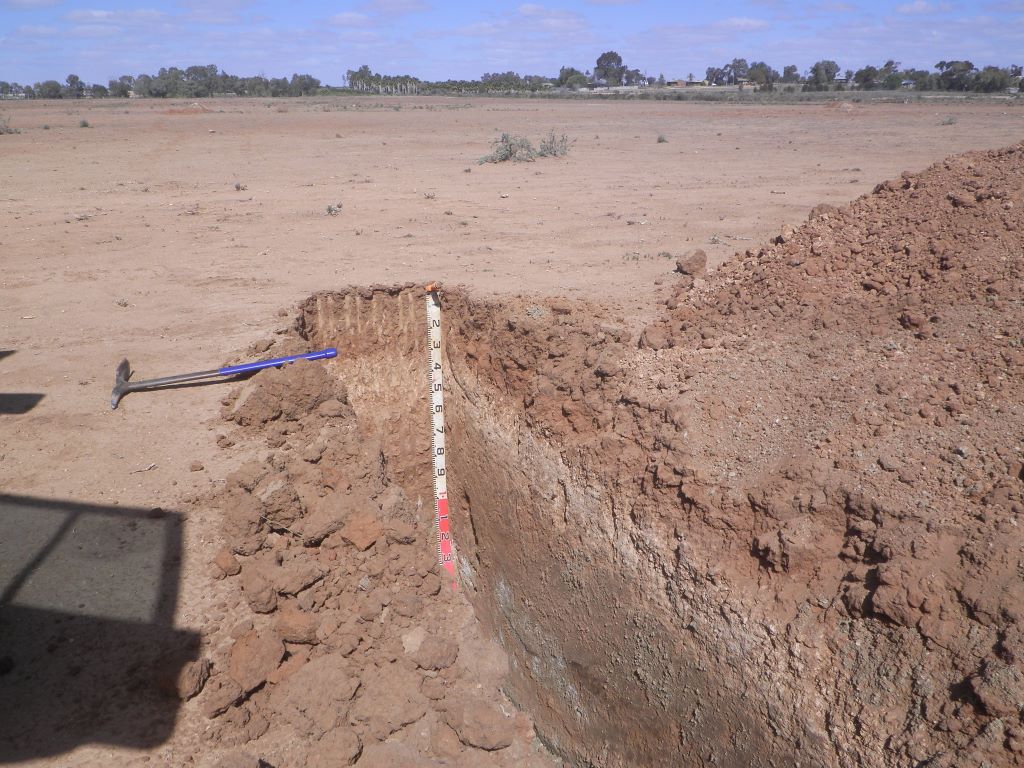
Figure 2. Soil pits are an excellent way to identify soil texture, root zone depths and drainage characteristics. Photo: Mallee CMA
Designing the irrigation system according to soil type will help achieve even growth, maturity, yield and fruit quality, allowing efficient irrigation management as each soil type and variety can be irrigated according to its individual requirements. For example, lighter soil types that need more frequent irrigations can be managed independently from heavier soil types where waterlogging is possible.
The results from soil moisture monitoring should reasonably represent the moisture content of each irrigation unit, and a well-designed drip system will help achieve this.
For more information see Soil survey for soil sampling for irrigated horticulture.
Irrigation system design
An irrigation system design is best left to a professional designer who can select the correct equipment to apply the right amount of water at the correct application rate for the soil. Growers should protect their investment by using an Irrigation Australia Limited (IAL) Agricultural Drip Micro Certified Irrigation Designer (CID). A directory of certified designers is available from Irrigation Australia.
A correctly designed irrigation system is essential. Experience has shown that systems are more likely to be successful if designed appropriately, installed correctly, and managed well. If any of these aspects are ignored, production potential may not be reached.
Pipeline material, size and class should be the most cost-effective, considering the initial capital cost and the long-term running costs. The design should comply with industry standards such as a maximum emitter discharge variation of ± 5% within a valve unit, and maximum pipe velocity requirements. The pump and motor should be selected for maximum efficiency, considering the size of the planting (current and future), topography and the maximum number of shifts.
The basic components of a drip irrigation system are outlined in Figure 3 below.
When planning a system, look at the quality and suitability of the components rather than choosing them on price alone.
If correctly designed, the drip irrigation system will be able to meet maximum crop water demand. Newly designed systems can usually do this. When expanding a system, especially when using existing pumps, the ability to keep up with crop water requirements may be compromised. Structure the initial design to allow for possible future developments.
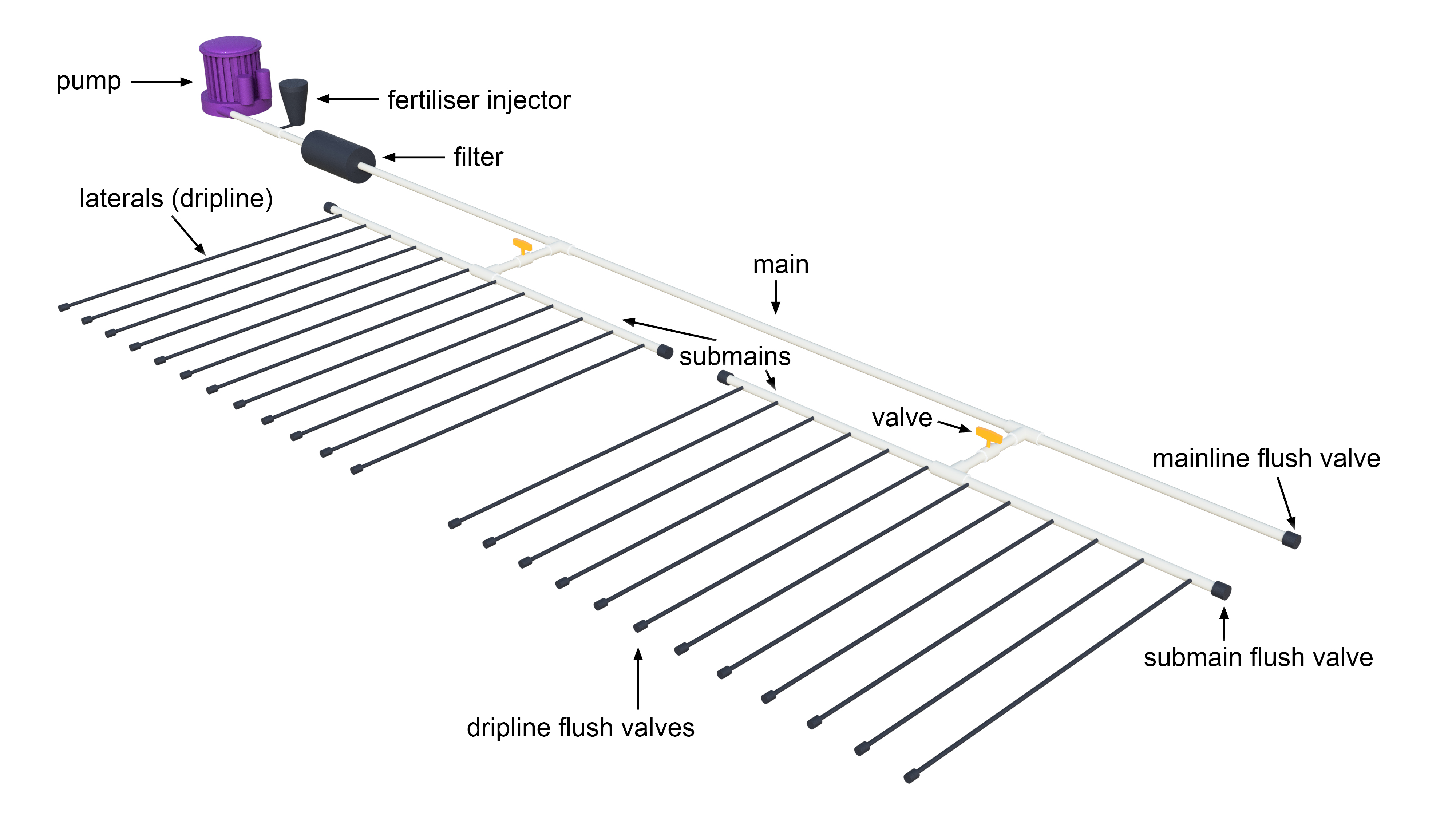
Figure 3. Typical surface drip system layout.
Maximum number of irrigation shifts allowed
‘Irrigation shift’ refers to the arrangement or aggregation of selected irrigation unit(s) of an irrigation system delivering water at a particular time.
Regardless of the size of the block or the irrigation management program chosen (e.g. pulsing), establishing whether an irrigation system will meet the peak water requirement of a crop can be achieved by determining the maximum number of shifts a system can run. This is a more critical question for drip irrigation than full cover sprinkler systems, due to drip systems having a lower application rate (full cover equivalent), therefore requiring a relatively longer run time to meet crop water requirements. The following equation is used to determine the maximum number of shifts possible for a given drip system and crop type.
Assumptions: | |
Maximum daily water requirement of crop = 8.0 mm/d | = 8.0 mm/d |
System application rate = 1.3 mm/h (full cover equivalent) | = 1.3 mm/h (full cover equivalent) |
Daily maximum hours pumping available = 18 h (25% pumping downtime) | = 18 h (25% pumping downtime) |
In this example, no more than 3 shifts are possible in order to meet peak crop water demand.
Many new drip irrigation developments now adopt low application systems (0.6–0.9 mm/hr) over larger areas, with a lower number of shifts (often a single shift).
Using the same daily crop water requirement (8 mm/d) with a low application system (0.9 mm/h), the following is obtained for comparison:
Low flow systems have smaller emitters and therefore require stringent maintenance programs. Visit the Drip maintenance and monitoring page.
More shifts can be run if irrigators are willing to pump 24 hours a day. While this is acceptable, it does not allow any flexibility to cover pump repairs following a breakdown nor allow for extra pumping during heatwaves (where daily water requirements would exceed 8.0 mm/d in the example above).
It’s important to ensure that every tree receives the right amount of water. A common issue can occur where plants at the ends of rows don't receive enough water because there are fewer emitters around them. This happens when the in-line drippers are connected to long blank poly offtakes (Figure 4) or when the laterals end too early at the last tree (Figure 5). In orchards running north-south in hot inland regions, the first or last tree on the northern edge often requires more water due to exposure to hot winds. This, combined with a reduced water supply from fewer emitters, can lead to poor tree health.
To ensure these trees receive adequate moisture, ensure that the drip line is run a little closer towards the headland, past the edge of the crop canopy, and use shorter offtakes from the submain and flushing manifold. If the offtakes are too long, another option is to insert button dripper(s) of the same discharge and spacing into the blank offtake (Figure 6).
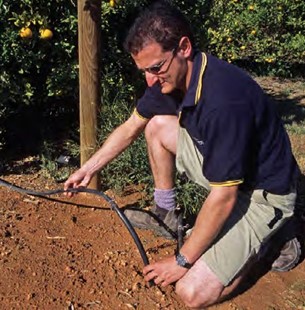
Figure 4. Offtakes can be shortened, and laterals extended to ensure the last tree in the row receives adequate moisture.
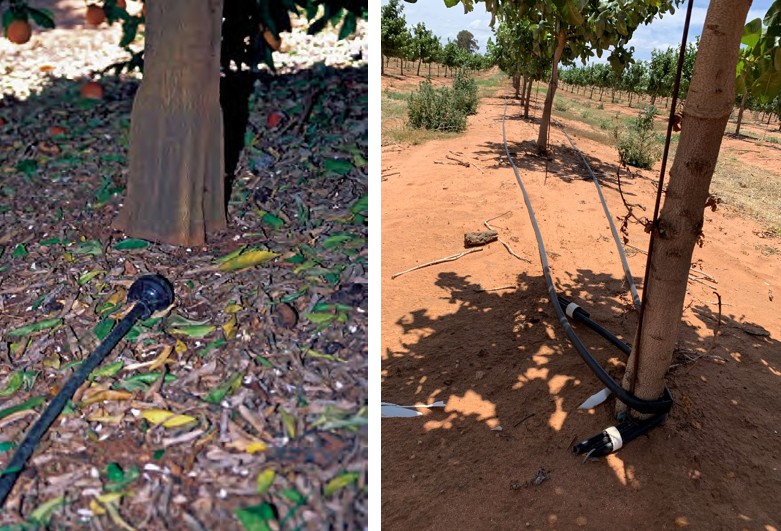
Figure 5. Ensure laterals are long enough to supply adequate water to the last tree.
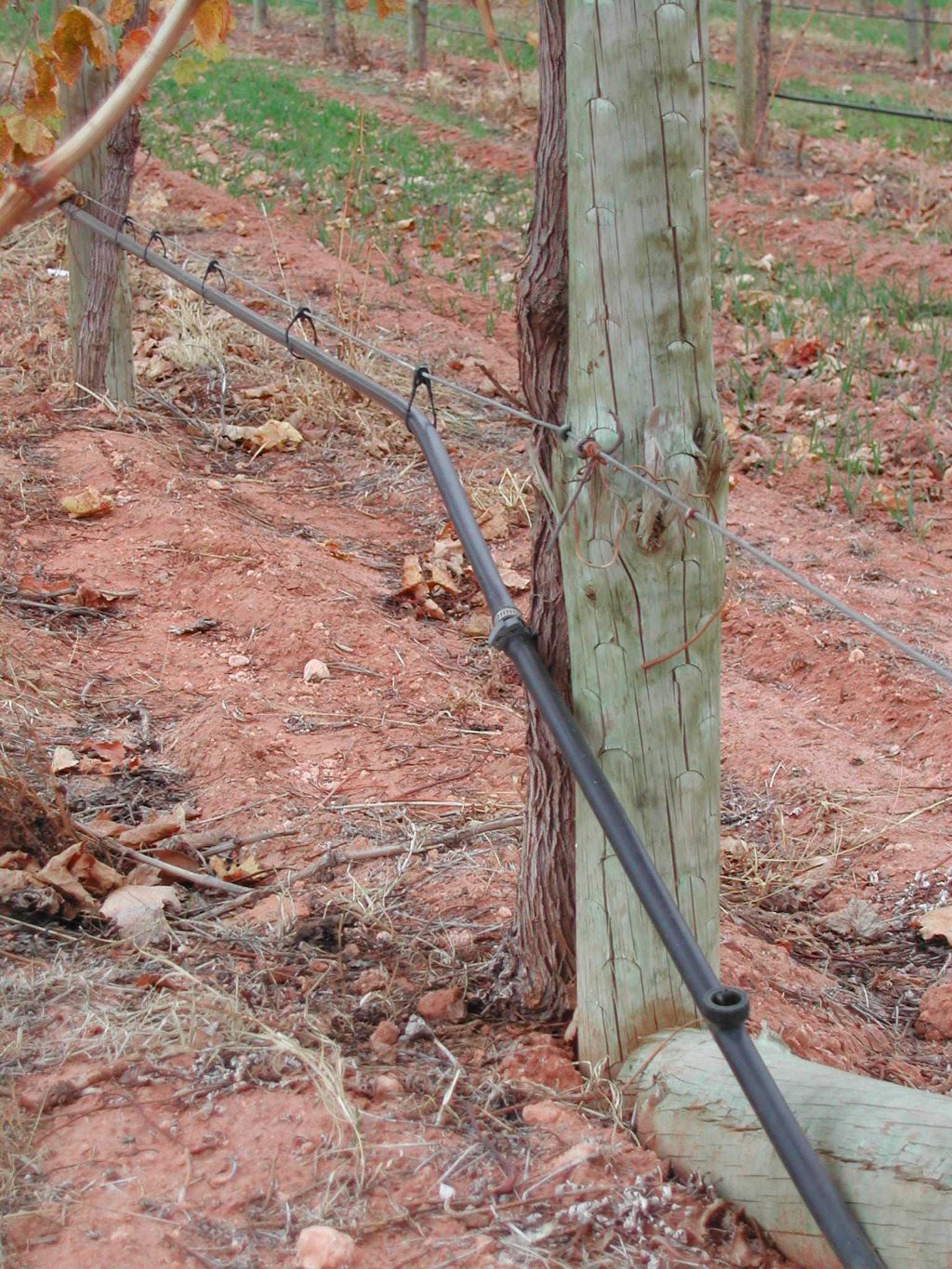
Figure 6. Button dripper added to a blind offtake ensuring the last vine receives adequate water.
Choosing an emitter
The emitter – or dripper - is the device attached to the drip line or lateral to control the rate of water discharge from the lateral. It is important to choose the right emitter for the irrigation system. Only the more durable (and generally more expensive) drippers and drip lines are suitable for perennial horticulture. Cheaper drip line is normally designed for single season use only, like vegetable crops.
When selecting an emitter, the main properties to consider are:
- Pressure compensating or non-pressure compensating
- Type of dripper, and specialty function, such as non-drain (ND)
- Dripper quality, including manufacturing characteristics and susceptibility to clogging
- Dripper discharge and spacing.
Pressure compensating or non-pressure compensating
Modern emitters can be classified into 2 types: pressure compensating (PC) and non-pressure compensating (non-PC).
PC emitters maintain a consistent discharge within a wide pressure range (between a maximum and minimum). They achieve this by using a flexible membrane or diaphragm that deforms with increasing pressure, restricting the flow path. However, PC emitters cannot increase the discharge if the pressure is too low (e.g., below 5 meters), but they can regulate it at high pressures.
On the other hand, non-PC drippers experience discharge variations with changing operating pressures. In a well-designed and properly operated system, the discharge of non-PC emitters should vary by less than ± 5%.
Hydraulic characteristic of non-pressure compensated drippers
The hydraulic characteristic of an emitter refers to the relationship between the operating pressure and the discharge rate.
In irrigation system design, it is important to ensure that the variation in discharge rate among all emitters stays within an acceptable limit, typically less than ±5% of the design discharge rate.
The hydraulic characteristic helps estimate the maximum allowable pressure variation along the lateral to maintain consistent discharge rates for individual emitters. This minimises the costs associated with piping and pumping while ensuring proper irrigation.
In the case of non-PC emitters, their specified discharge rate (e.g., 2 L/h) is considered the nominal discharge rate at a specific pressure, typically 100 kilopascals (kPa). If the pressure deviates from this specified pressure, the discharge rate of the emitter will also vary (Figure 8).
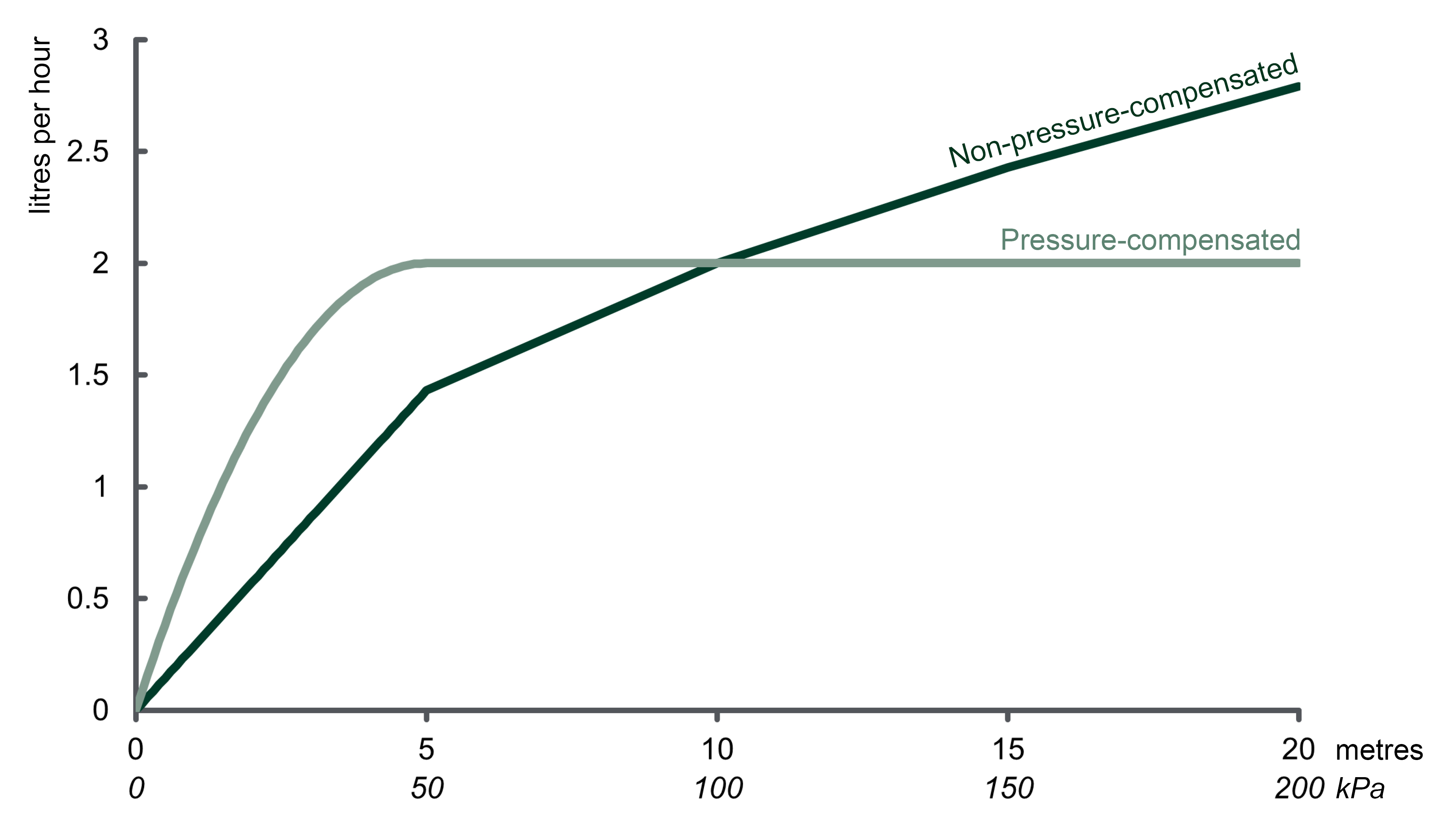
Figure 8. Discharge versus pressure of 2 L/hr pressure compensated and non-pressure compensated drippers.
Actual discharge rate (L/h) | |||||
---|---|---|---|---|---|
Nominal flow rate (L/h) | 50 kPa | 100 kPa | 150 kPa | 200 kPa | |
1.3 | 0.94 | 1.3 | 1.56 | 1.79 | |
2.0 | 1.43 | 2.0 | 2.43 | 2.79 | |
3.0 | 2.15 | 3.0 | 3.64 | 4.18 |
Table 1. Pressure and flow rate variation in a common non-pressure-compensating drip system. Source: Adapted from Netafim Australia brochure, Dripline 2000–2025 multi-seasonal dripper line.
The emitter discharge exponent (x) describes the relationship between pressure and discharge, indicating the sensitivity of the emitter discharge rate to pressure differences (see Table 2).
The value of x is typically between 0.0 and 1.0. The greater the value, the more sensitive the discharge is to pressure changes. Generally, an emitter discharge exponent of 0.5 or less is acceptable for non-PC emitters used in permanent horticulture. A dripper with a large exponent (for example, x = 0.8) has greater sensitivity to pressure variation than one with a smaller exponent (for example, x = 0.4).
A value of 0.0 means that the discharge rate will not change with pressure. PC drippers have very low x values (in theory 0.0), but generally do show some minor discharge variation in practice.
Table 2 shows that for a 20% change in pressure (due, for example, to friction losses or elevation changes), drippers with x = 0.4 only have a 7.6% change in discharge (see highlighted cells in Table 2). If the same pressure change was to occur for a dripper with x = 0.8, the discharge would change by 15.7%.
The emitter discharge exponent (x) should be available from all drip manufacturers but is rarely available for drip tape or single-season products.
Emitter discharge exponent (x) | |||||
---|---|---|---|---|---|
0.4 | 0.5 | 0.6 | 0.7 | 0.8 | |
% pressure change | % discharge rate change | ||||
10 | 3.9 | 4.8 | 5.9 | 6.9 | 7.9 |
20 | 7.6 | 9.5 | 11.6 | 13.6 | 15.7 |
30 | 11.1 | 14.0 | 17.1 | 20.2 | 23.3 |
40 | 14.4 | 18.3 | 22.3 | 26.6 | 30.9 |
50 | 17.6 | 22.5 | 27.5 | 32.8 | 38.3 |
Table 2. The change in percent discharge rate of emitters with various emitter discharge exponents.
Source: Burt and Styles 1998.
Both PC and non-PC drippers use turbulent flow, where water moves through the dripper labyrinth in a very unorganised way due to the internal ‘teeth’ design of the flow path (Figure 9). Turbulent flow mixes the water, causing it to maintain a high velocity on the dripper wall, sweeping clay and silt particles from the wall into the centre of the pathway and out of the dripper, preventing any build-up or blockages from sedimentation.
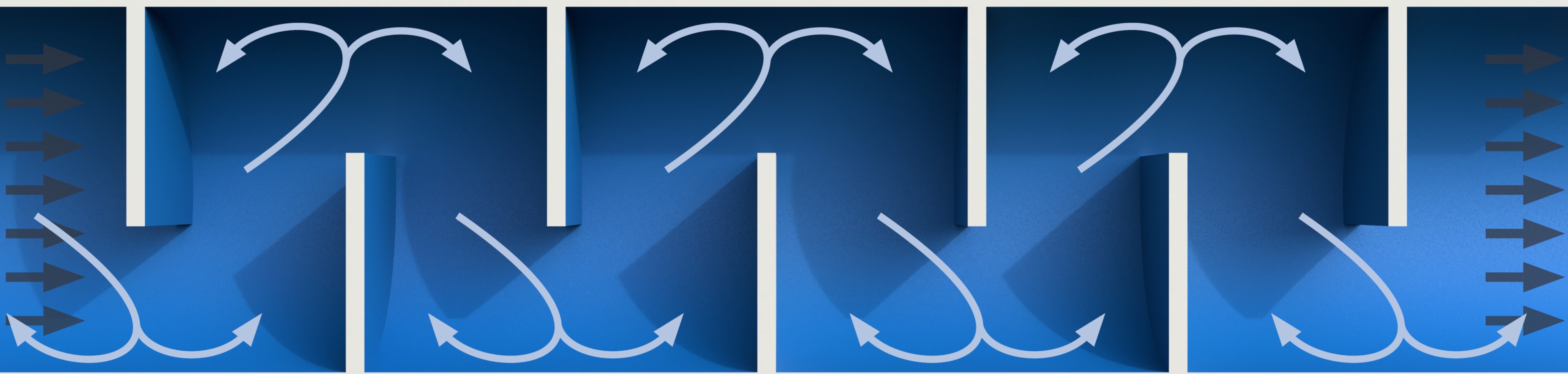
Figure 9. Turbulent flow pattern.
Turbulent flow drippers are produced by all major irrigation equipment manufacturers to suit a range of operating conditions (Table 3). All turbulent flow emitters have widely varying performance characteristics and are suited to different applications. Drip irrigation system designers should be aware of these performance characteristics when choosing an emitter for a particular situation to ensure the irrigation system fulfils the basic requirement of uniform water application to the crop.
Types of drippers
Various emitter types are available, and the most common types are outlined here:
Dripper | Description | Comments |
---|---|---|
Online drippers |
|
|
In-line drippers |
|
|
Non-drain (ND) emitters |
| Suitable in:
|
Anti-suck-back (AS) emitters |
| Highly suited to:
|
Anti-root penetration |
|
|
Table 3. Types of drippers
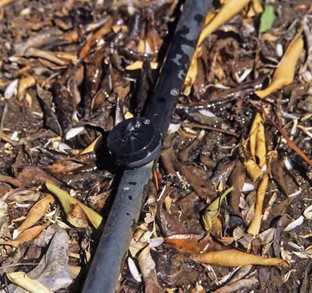
Figure 7. Online button dripper inserted into blank poly pipe.
Dripper quality
The small size of the emitter flow path (typically 0.5-1 mm diameter) requires precise manufacturing. Even small deviations in size can significantly impact the discharge rate.
Ideally, each emitter of the same type would have identical performance and the discharge rate of any emitter along a lateral could be predicted for any given operating pressure.
Tests on various emitter types have shown that most emitters have discharge rates within 2-10% of the average measured rate. However, there have been cases where variations larger than 20% were observed. Such variations would result in inconsistent water volumes, with some trees receiving excessive water while others receive inadequate water.
Coefficient of variation: new drippers are tested by manufacturers to determine how much variability exists in manufacturing. The measure of manufacturing precision is called the coefficient of variation (Cv, see Table 4) and is a measure of the difference in discharge rate of a group of emitters at the same pressure. This measure should also be available from retailers upon request.
Classification | Cv |
---|---|
Excellent | less than 0.03 |
Average | 0.03–0.07 |
Marginal | 0.07–0.10 |
Poor | more than 0.10 |
Table 4. Emitter coefficient of variation (Cv)
Pressure compensating drippers, due to their relatively complex construction, tend to have a large manufacturing Cv compared to non-pressure compensating emitters.
Another measure that some manufacturers use, particularly for drip tape or single-season products, is emission uniformity (EU%). Emission uniformity incorporates the flow rate variations from both manufacturing (Cv) and pressure variation to estimate irrigation efficiency. The recommended EU is greater than 90%. The calculation of EU is complex and requires knowledge of the emitters Cv. Some manufacturers provide online calculators to determine the EU. Emission uniformity is used by manufacturers for determining the maximum length of a drip line on flat ground given a certain operating pressure and emitter spacing. None of these measurements are interchangeable. An EU of 90%, for example, does not relate to a flow variation of 10%. For more information see Drip system maintenance and monitoring.
Qmin (L/h) = lowest emitter discharge, Qave (L/h) = average emitters discharge, Cv = coefficient of variation, n = number of emitters per plant.
Clogging susceptibility
Two features of drippers that determine how readily they remain clean and functional are the filtration area and labyrinth size (width, depth, and length).
Both features are measurable and should be readily available from dripper specification sheets. Equations are available using turbulence coefficients and effective filtration to provide comparison of dripper clogging susceptibility between products.
Dripper discharge
Dripper discharge should not exceed the soil’s infiltration rate. Operating below this infiltration rate will minimise the saturated area immediately under the dripper and avoid run-off.
Traditionally a dripper discharge of 2.5–4.0 L/h was considered suitable for lighter soil types, and a discharge of 2 L/h was used in heavier soil types to minimise surface run-off and ponding, promoting deeper water penetration. Today dripper discharges generally lie between 1–2 L/h regardless of soil type, combined with a relatively closer dripper spacing.
Dripper spacing
Soil texture also traditionally determined the appropriate dripper spacing. Drippers should be spaced to create a continuous wetted strip along the tree row. In heavier soils, a relatively wider spacing was originally considered sufficient, with closer spacings required in lighter soils to establish this continuous wetted strip.
Closer spacings (0-3-0.5m) are now becoming a common trend in irrigated horticulture, regardless of soil type. This achieves a better wetting pattern in a shorter run time. Some manufacturers can produce drip line with very specific emitter spacings (eg 0.48m) upon request.
Dripper discharge, spacing and soil type
Soil type should still be a factor when determining dripper discharge and spacing. Discharge and spacing affect the wetting pattern in the soil and determine the application rate, which influences how fast water moves into the soil.
Drip irrigation is based on a network of drippers that should distribute water and fertiliser in a uniform pattern to each tree. Although the system can deliver water uniformly, the soil profile might not be wet uniformly.
Immediately under each dripper the soil is saturated, with little air available for plant roots. Correct selection of discharge and spacing can minimise these saturation areas.
Between the saturated zone and the wetting front there is a zone of balanced water and air content (Figure 10). This is suitable for plant uptake of water and nutrients and the volume of this area should be maximised for optimum plant performance.
To use drip irrigation efficiently, a continuous wetted strip along the tree row must be created.
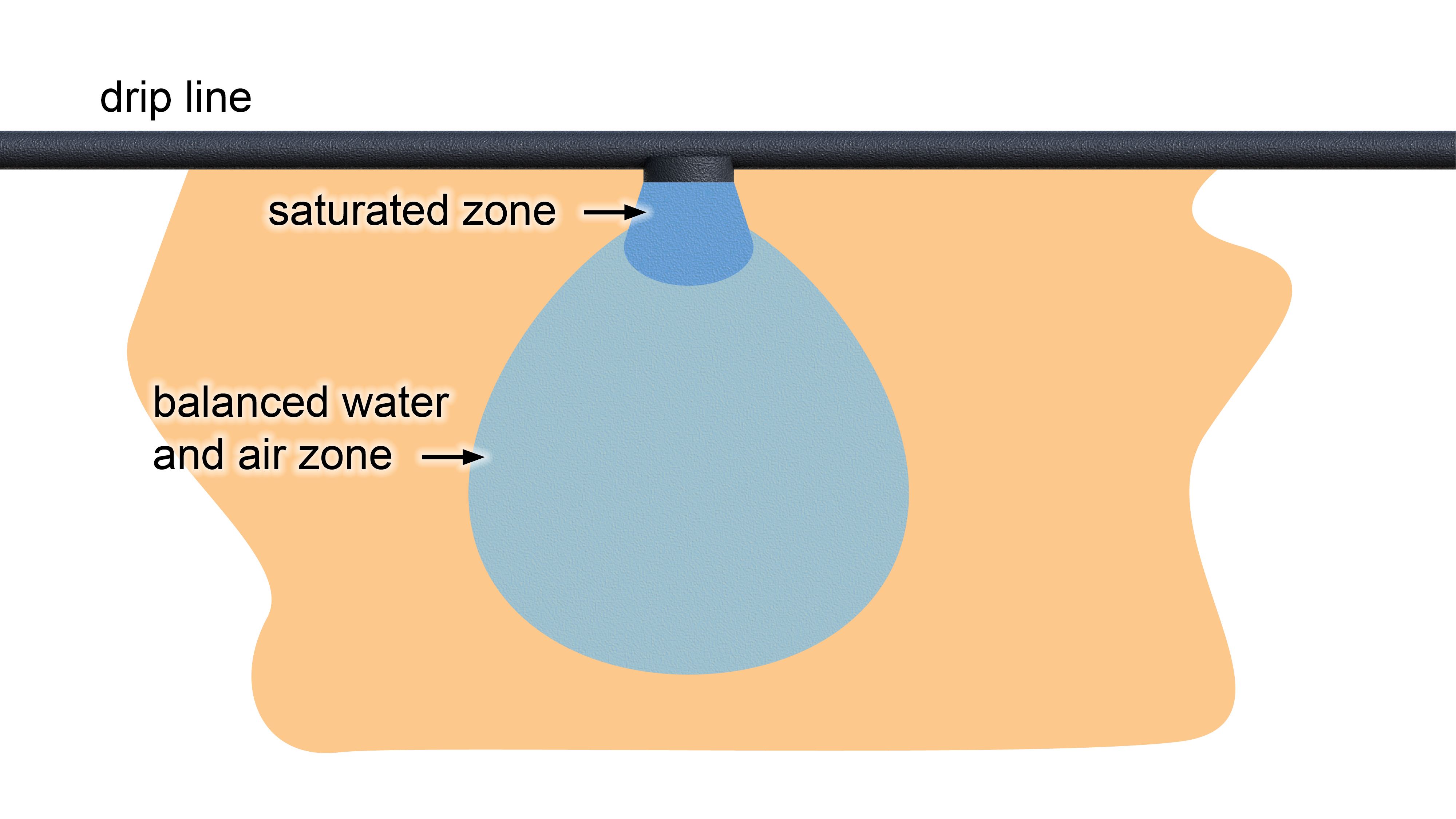
Figure 10. Typical drip soil-wetting pattern.
The wetted strip may not be visible on the soil surface between drippers, but it should be around 30 cm below the surface Here the trees can develop a dense root system along the row, using the maximum wetted area that can be made available by the laterals. Saline fronts between emitters along the row are eliminated and the root system will be larger, more efficient, and less vulnerable to water and nutrient stress, generally resulting in healthier trees (see Figure 11).
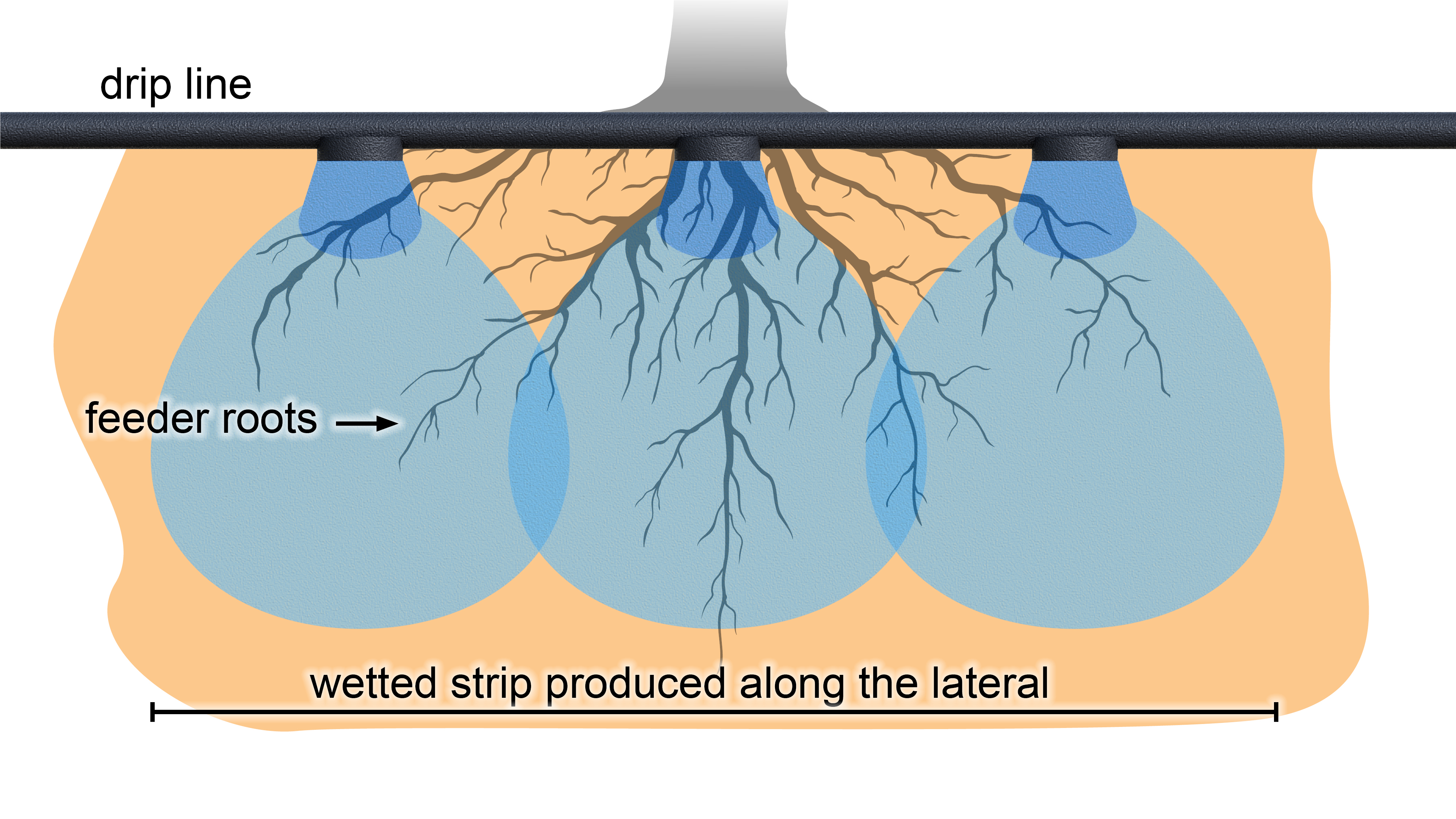
Figure 11. Cross-section along a row showing wetted strip.
Soil type dictates the wetted pattern underneath each dripper. In general, sandy soils have large pores which allow water to move freely downward due to gravity. There are only a few smaller pores, and this limits the sideways spread from capillary action. In sandy soils, the wetted patterns tend to be long and narrow.
Heavier clay soils have numerous small soil pores and few larger ones, meaning the water tends to spread sideways and cannot move down freely. In these soils, the patterns produced tend to be shallower and wider for the same volume of water applied (see Figure 12).
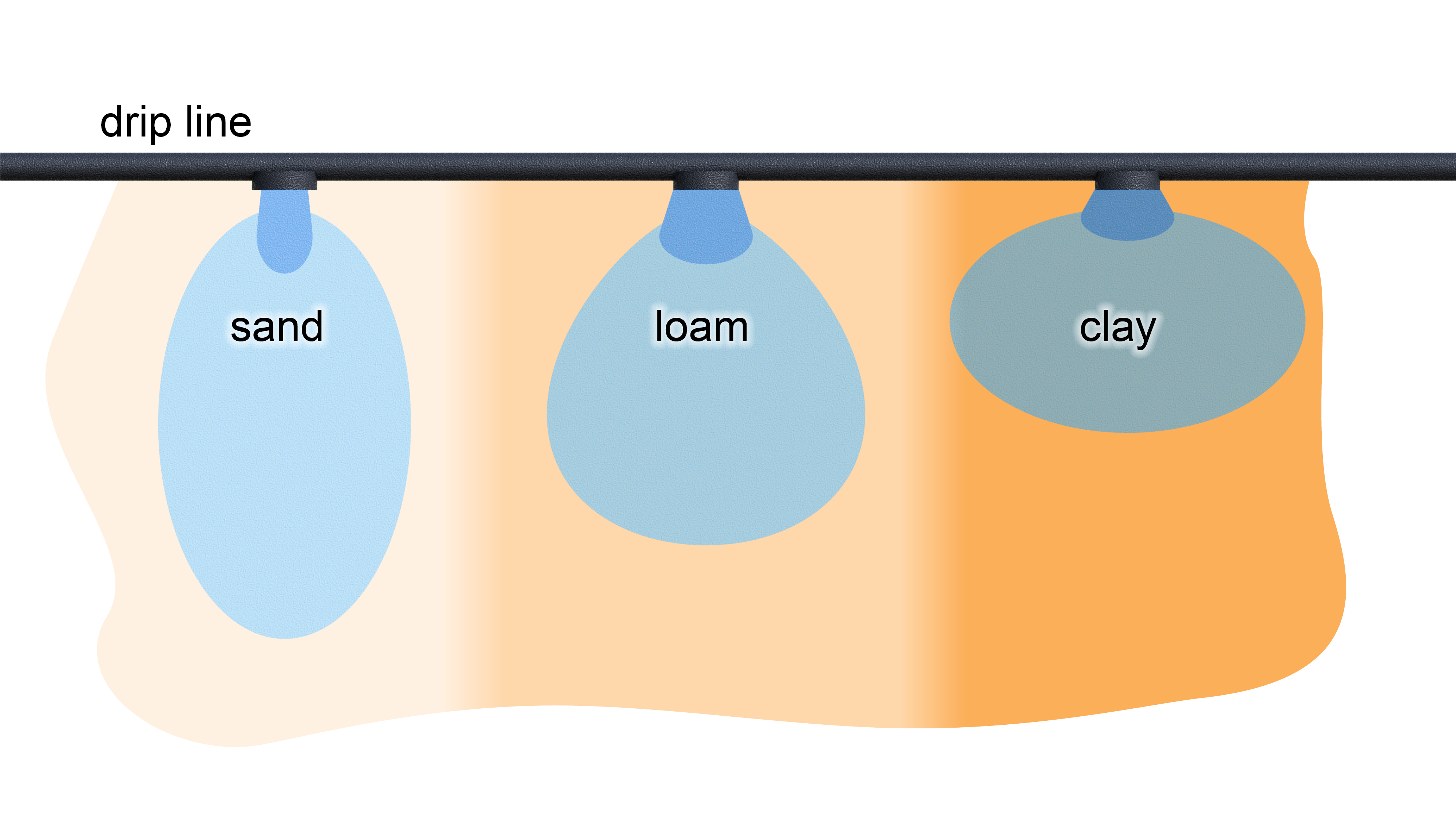
Figure 12. Dripper wetted patterns in different soil types.
However, this generalisation is not true when heavy soils have dried out to such a level that cracks develop in the soil. Once cracks develop, wetted patterns in clays initially tend to be long and narrow, similar to that produced in sands.
The length of irrigation and dripper discharge also determines the size and shape of wetted patterns. A longer irrigation produces a wider wetted pattern for a given dripper discharge and soil type. High discharge drippers produce a wider zone of saturation under the emitter, assisting lateral movement and resulting in a wider wetted pattern for a given irrigation time and soil type.
Pulsing is a drip irrigation management practice which many growers use. Pulsing involves setting up the irrigation controller to turn an irrigation shift on and off (say one hour on, one hour off) throughout an irrigation period. The most commonly claimed benefit is that it encourages greater lateral water movement. A non-drain drip line is ideal for this situation (See drip drainage section below).
Drip application rate
Application rate is the term used to describe the rate at which water is applied to the soil. It is usually measured in millimetres of water applied per hour (mm/h). It relates to a volume of water applied to a given area, and in drippers it is determined by dividing the volume of water discharged from a single dripper into the area that the dripper covers (the product of their spacing).
Assumptions:
Oxygation
All irrigated crops can be exposed to saturated soil under certain conditions. This is especially the case for crops which are drip irrigated as water is delivered to the soil from a point source creating sustained wetting fronts, particularly in heavy soils of low permeability. This scenario has been termed the ‘irrigation paradox’; where irrigation is applied to meet the plant’s water requirements but at the same time purges air and therefore oxygen out of the rootzone, potentially affecting root activity.
Alleviating this low oxygen environment can be attempted with the use of aerated water for irrigation, increasing oxygen availability in the rootzone. Oxygation is the term used for aerated irrigation water applied through drip irrigation and has been shown to benefit the growth of several crops, particularly in heavier soils.
Oxygen can be added to the irrigation water through a range of methods, including installing simple venturi systems, where the amount of air ingress will depend on the pressure differential across the venturi, or more complex systems such as nano-bubble generators and oxygen injection. Low-rate continuous injection of hydrogen peroxide has also been used to provide oxygen to rootzones.
The most likely scenario when this practice could be considered is with SDI. The benefit is less effective with surface drip irrigation, and ineffective when drip line is suspended above the ground.
Drip line location
Vine crops have one drip line along the vine row, while mature orchards typically have 2 drip lines along the tree row on both sides of the tree. If a third drip line is introduced between these laterals along the butt line (or if the outside laterals are located too close to the butt), care must be taken to avoid problems such as root rot in susceptible crop types.
If developing a drip irrigation system for an orchard, the system should be designed as a 2-drip line system (or 3 as described above), with a single line installed initially to support the young trees (Figure 13).
Trees should be planted directly next to an emitter at spacings consistent with the drip line emitter spacing (such as tree spacings of 1.5 or 2.0 m for emitters spaced at 0.5 m).
This ensures that an emitter is located directly on top of the undeveloped root zone in the first year of planting, supplying adequate water and nutrient to the young tree.
The second line does not initially need to be installed, but if necessary, can be used to grow a mid-row crop to provide some protection from wind or sand damage. Sorghum (Figure 14) or Sudax are commonly grown in these situations. Often a cheaper one or 2-season drip line is used, as it can be difficult to remove the hose from a mature stand of sorghum without damaging the drip line.
In the second year the original drip line used to irrigate the trees is usually moved along the row, moving emitters away from the tree butts to prevent root rot problems. This line only has to be moved half the length of the emitter spacing, i.e. 0.25 m for emitters spaced every 0.5 m.
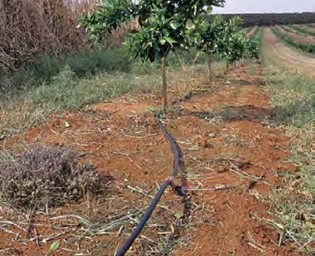
Figure 13. A single drip line can provide adequate water for young citrus trees.
In the third year the second drip line is installed closer to the trees with each drip line now positioned approximately 0.25 m on either side of the tree. In the following years both drip lines are moved away progressively from the tree line to avoid the trees experiencing dramatic changes to the wetting pattern, while at the same time helping to develop a strong root system to support and anchor the mature tree. The drip lines should finally be positioned 0.5–0.75 m on either side of the tree butts, depending on soil type.
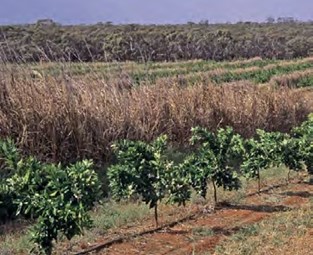
Figure 14. A sorghum crop providing protection to young citrus trees.
For many mature crops it is critical that the drip line does not move significantly throughout the season. Water and fertiliser distribution within the soil, as well as salt movement, should be maintained, allowing a healthy root zone to develop below the drip line. To ensure this occurs, drip lines might need to be staked along the row (Figure 15).
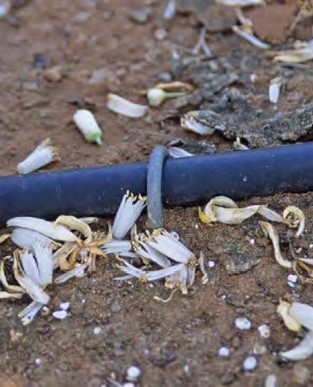
Figure 15. Staking a drip line.
When trees are grown on mounds, a closer drip line spacing is often needed to keep the drip line on top of the mounds. This is generally fine because mounds have well-drained soil that is less prone to waterlogging. Staking the drip lines on each side of the mound near the tree prevents the line from sliding off the mound and keeps it at a safe distance from the tree base to prevent root rot.
Drip irrigation in interplant situations, where there are both young and mature trees, presents challenges. Young trees have small root zones, requiring the drip line to be close to the tree base. Mature trees, on the other hand, need the line to be positioned 0.5-0.75 metres away from the base to avoid root rot issues. One solution is to loop the drip line along the tree line in the early seasons until the young trees have matured. (Figure 16)
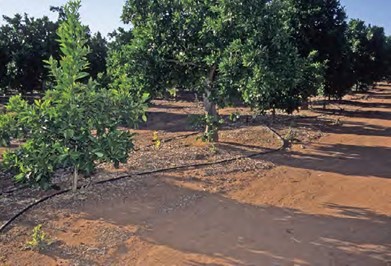
Figure 16. Looping a drip line close to young trees but further away from the mature trees.
Drip drainage
Drip system drainage or drain-out occurs following each irrigation event when the remaining water in submains and laterals continues to run inside the pipework to the lowest point(s) in the orchard and is emitted. Studies have found that emitters in these low areas can emit water continuously between 60-minute irrigation pulses and continue for as long as 24 hours following complete irrigation shutdown.
Drain-out is a concern, especially in large systems with long pipes, frequent irrigation, or pulse irrigation. The more frequent the irrigation event, the more frequently drain-out occurs following each system shutdown/turnoff. There is an increasing level of interest in minimising or eliminating drip drainage. New drip irrigation designs should consider drip drainage at the design stage, particularly if pulse irrigation is being considered. Retrofit options also exist. Options to reduce drip drainage include:
- use of non-drain (ND) drip line
- installation of non-return valves in submains running uphill
- installation of sustaining valves in submains running downhill
- non-leakage valve installation in drip lines
- relocation of valves.
For more information see Drip drainage.
Sub-surface drip irrigation for permanent horticulture
Intermittent interest in sub-surface drip irrigation (SDI) in permanent plantings exists in Australia. There are advantages and disadvantages of SDI in permanent horticulture.
Advantages of SDI are:
- water is delivered directly to the root zone
- less surface water for weed growth
- reduced evaporation from the soil surface.
Actual water savings are not well established There is some thought that if the drip line is below the surface, the soil surface below the canopy will be more barren, exposed to greater temperatures, and that water demand from trees will increase, offsetting the reduction in water use from surface evaporation.
Interest in SDI is often driven by non-water related issues such as removing the drip line from interfering with harvesting nuts from the orchard floor, or mechanical weed control necessary for organic growers.
Placing the drip line below the surface unfortunately creates a new set of challenges. The main disadvantage is that blockages are difficult to detect and locate. Direct observation of the dripper discharge rate is not possible, and it is difficult to evaluate the system and measure the system uniformity. Accurate water meters are therefore essential with SDI to measure application rates and flag blockages or leaks. Blockages may be very difficult to locate until it is too late, and trees begin to show signs of stress. The use of spatial imagery to identify any drip line blockages or leaks is highly applicable for SDI (See Alternative monitoring methods).
Maintenance programs (flushing and disinfestation) need to be carried out more frequently than for surface drip irrigation. Repairs and maintenance costs should also be factored in if considering SDI. Soil may have to be excavated 30cm deep and 2m long before a suspected leak or blockage is found. Frustration can result once a leak is fixed and the system repressurised, only to find another leak exists a little further along.
Blockages can be due to the standard reasons which exist for surface drip irrigation, with SDI additionally prone to blockages due to root intrusion, root pinching, and soil suck-back. In addition, the temperature of irrigation water may drop a few degrees when moving from above to below ground. If dissolved elements such as calcium exist in the water this can precipitate, creating mass blockages in the drip line (See water quality section above).
Root intrusion
SDI systems potentially have the serious problem of root intrusion, particularly when the surrounding soil is dry and crops are looking to scavenge any available water. If herbicide injection to control root intrusion is required, a permit or label extension may be necessary depending on specific regulations.
Modern drip lines are available with chemical (copper) impregnated into the dripper to inhibit root intrusion. It is generally accepted that this may postpone or delay root intrusion, but not completely eliminate it. Root intrusion can also be controlled with acid injection, depending on how severe the intrusion has become.
Even if root penetration is avoided, there is no known method of avoiding drip line pinching, which can occur in vigorous root systems.
Vacuum in laterals
When irrigation ceases in SDI, and the system is shut down, drainage continues in the laterals and sub-main. This can cause a vacuum effect in the laterals, resulting in soil particles being sucked into the dripper orifices, causing blockages. To avoid this, air inlet vacuum relief valves must be installed at the end of each sub-main and flushing manifold, as well as at the high points of the laterals. Non-suck-back drip line products are recommended in SDI situations (see Types of drippers section above).
Insects and rodents
Other problems have been encountered with various insects biting small holes in the laterals, causing seepage, and mice chewing holes in search of water. Standard measures should be used to control these pests. Damage often becomes a particular problem if the system has laid dormant for any length of time (over 6,500 holes reported due to marsupial mice in an installation that lay unused for 2 years due to drought). New drip products claim to deter insect damage by having insecticide embedded directly into the drip line.
Installation
Installing the drip line will require a tine that can carry the drip line into the soil. Depending on the soil type and tractor power, the depth of the lateral can vary. Installation depth should realistically be no more than 30 cm, although limited recommendations are available for permanent horticulture. Some users prefer the drip line as close to the surface as possible. These irrigators are primarily installing the drip line below the surface to avoid damage from machinery such as slashers, and water reaching the surface is not of concern. Installation depth will be a compromise between supplying sufficient moisture to feeder roots near the surface and avoiding excessive water coming to the surface (channelling).
In young orchards, drip line is generally left on the surface for a few years, close to the butt, to enable water to reach the immature rootzone. The drip line can be installed in the correct sub-surface location required for mature plantings once the trees are established (3–4 years old). Installation in large mature plantings is difficult as the larger tree canopy (unless heavily pruned) does not allow the installation equipment to install drip lines at ideal distances from the butt of the tree (see Drip line location section above). Damage to rootzones can also be a concern. Therefore, SDI installation in mature trees must be carefully considered.
Several brands of drip line have been used in trials and most reputable brands have proven to be reasonably successful for SDI. AS emitters are necessary, while ND emitters should be avoided. Installing emitters facing up improves lateral water spread.
While SDI is potentially the most efficient system available, growers need to weigh up the disadvantages of such a system before adoption. With continual improvements in management and technology, SDI could become a more favourable option in the future.
Frost control and drip irrigation
Lack of frost control is often viewed as a major disadvantage of drip irrigation. Growers in frost-prone areas have historically preferred using low-level or overhead sprinkler irrigation. If converting to drip irrigation from overhead sprinklers in this situation, seriously consider retaining the overhead system, at least in likely frost pockets.
Low-output frost protection heads can be an option in some instances where drip irrigation already exists. These sprinklers generally operate between 150 to 200 kPa: a pressure that is compatible with most drip systems. The sprinklers are usually mounted on risers above the canopy (Figure 17). Some models (such as flippers) apply water along the tree row only. In these situations, mid-row areas remain relatively dry, meaning these sprinklers can be used to control many more frosts than conventional overhead sprinklers, which can result in waterlogging. These sprinklers also enable a larger area to be frost-protected for a given flow or sub-main size compared with conventional sprinkler systems.
Given the value of water and other issues, frost fans are increasingly being adopted to overcome frost issues in perennial horticulture.
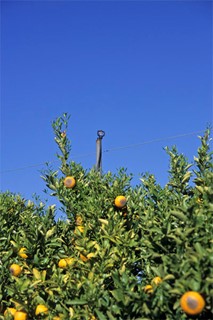
Figure 17. Low output overhead sprinklers may be an option for frost pockets or sensitive varieties that are currently drip irrigated.
Heat control and drip irrigation
Growers of heat-sensitive crops have also historically been hesitant to adopt drip irrigation, preferring full-cover sprinkler systems (above or below canopy). Purpose built cooling systems, mostly above the canopy (Figure 18), are increasingly being adopted along with drip irrigation in heat sensitive crops. A dual system therefore exists. The cooling system is designed to operate at a similar pressure and flow rate to drip irrigation and is compatible with a drip irrigation design. Cooling systems are operated to reduce temperatures and or increase humidity when conditions require, potentially avoiding damage to crops. These systems have also been used successfully for frost control.
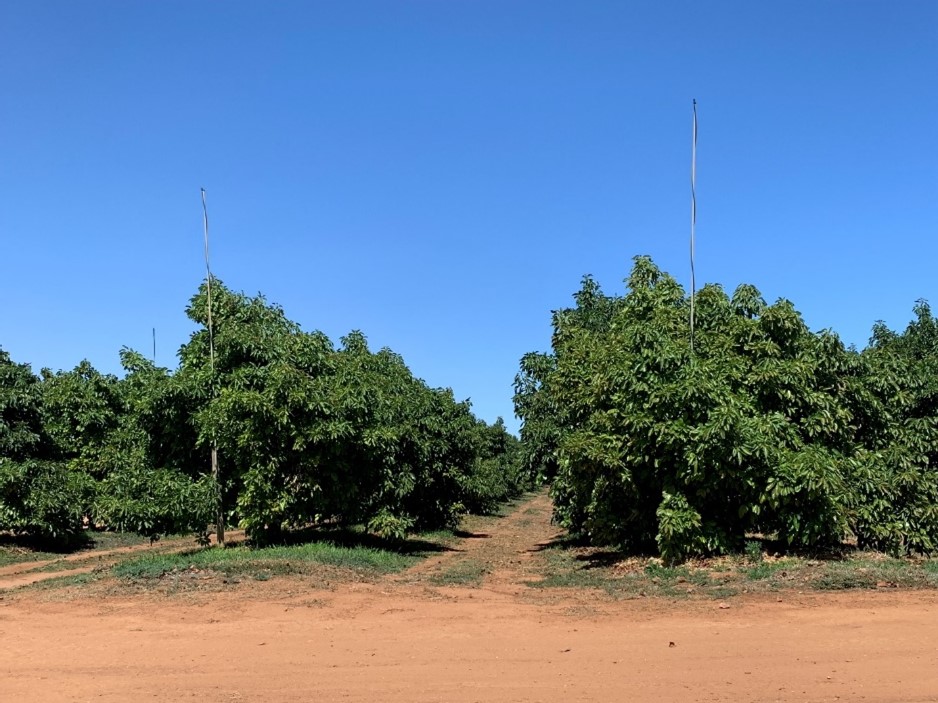
Figure 18. An overhead cooling system on a drip irrigated avocado crop.
Converting existing plants to drip irrigation
Irrigators are often cautious when converting mature trees or vines from a full cover irrigation method to drip irrigation. Some crop types (and rootstocks) are more sensitive to this conversion than others. Where an established orchard or vineyard is converted to drip, poor crop health and reduced productivity can occur in the first year after conversion if appropriate management practices are not adopted.
Poor tree health, most commonly observed in older trees or trees growing on shallow soil, is often due to a poor understanding of the different management practices the new drip system requires. The most common issue is that irrigators new to drip irrigation often do not initially recognise that more frequent irrigations are required.
The key aim when converting existing trees from full cover to drip irrigation is to quickly encourage enough root growth in the soil volume wetted by the drippers to support the plant during periods of peak water demand.
In some regions salt could have also built up under the tree, where the drippers and soon to be active rootzone are now located. This is particularly the case with furrow and overhead systems, and in un-skirted below canopy sprinkler situations. This salinity needs to be leached quickly. As drip applies water to a relatively small area compared with full-cover systems, the leaching power of drip systems is considerable.
To leach salts away from the tree row, the first few irrigations with drip should be reasonably heavy, considering crop water requirements, water tables and problem areas. Subsequent irrigations need to be scheduled to ensure under-watering does not occur. If the previously existing full-cover system is retained, be aware that frequently using this system could re-mobilise soil salinity back into the dripper-wetted profile.
An ample water supply is usually recommended in the first year after conversion. Growers should not be too concerned about slightly over-watering in the first year. A healthy orchard that successfully overcomes the conversion to drip irrigation can be efficiently drip-irrigated for many years. Applying ample water in this first season also encourages a greater lateral spread of water, which helps minimise the significant change in the new wetting pattern produced when drip irrigation is installed.
To encourage root growth within the wetted strip, it is important to apply soluble fertiliser through the irrigation system (that is, fertigate) early in the season. Use fertiliser high in phosphorus and nitrogen, as this will encourage the development of roots within the wetted strip. Ample phosphorus might exist in the mid-row area, but under drip irrigation, much of this becomes unavailable to the tree. Side banding fertiliser along the proposed drip line location in the season before conversion might also be an option.
When applying fertiliser, time the application over the whole irrigation period, allowing time to flush it from the irrigation system. It is generally recommended to apply nitrogen and phosphorus fertilisers weekly throughout the first season of conversion.
A nematode test and a compaction assessment are also recommended for sensitive crop types. If existing root systems are unhealthy due to nematodes, root rot or compaction, conversion to drip irrigation alone will not correct these problems.
The best time to convert to drip irrigation is normally straight after harvest, as this gives the plant extra time to adapt to the new system without a crop to support. Successful conversions have occurred during less-than-ideal periods, with trees carrying heavy crop loads, but in these situations, the risk of problems occurring is much higher, particularly due to early heatwaves.
During the first season, no stress should be observed as long as:
- ample irrigation is supplied to maximise lateral spread and leach accumulated salts
- a good fertigation program is adopted.
When converting to drip from an existing pressurised system, the tendency is to use existing pipework where possible. Discuss this with the irrigation designer. Often the effort needed to continue to use this pipework is not worth the small saving in capital cost. Existing asbestos cement submains are unsuitable for drip conversions because they tend to flake, and block emitters when disturbed during installation and once fertigation or injection processes begin. Retaining asbestos cement mainlines can be more acceptable if back-up filters are installed at all valves and, if acid injection is practised, the pH of the water is kept above 4.5.
Silt and clay build-up in the existing pipework can also render a new drip system ineffective by creating emitter blockages. If this is likely, it is again important to install back-up filters at each irrigation valve (see Filtration for drip irrigation) and to fully flush the system following installation.
It is highly recommended that soil moisture monitoring be adopted at the same time as the new drip system. This will readily identify the irrigation management program required under the new system. Good soil moisture must be maintained in these smaller wetted volumes created by drip irrigation. See the Converting mature citrus from full cover to drip irrigation fact sheet on the NSW Department of Primary Industries website.
Further information
Contact Jeremy Giddings: jeremy.giddings@agriculture.vic.gov.au
Acknowledgements
- Peter Henry, Agronomist, Netafim Australia
- Trevor Sluggett, Agronomy Manager, Nutrien Water, Renmark SA